Reach 2024: How does the brain give rise to the mind?
By Tyler Irving
From AI to optogenetics, new techniques and strategies are enabling researchers to learn from cognitive disorders and probe the root of consciousness
Every waking moment, something remarkable happens to you. Somehow, approximately three pounds of neurons, glia, proteins and other bits and pieces inside your skull produce the thoughts, feelings, memories and actions that define who you are.
How exactly physical matter gives rise to consciousness — often called the “mind-body problem” — is a long-standing mystery of both philosophy and science. It’s hard to assess how close we are to solving it, but what is clear is that new techniques and strategies are giving rise to a particularly rich period in consciousness research.
This research opens pathways to understanding cognitive functions, as well as new potential treatments for cognitive disorders such as Alzheimer’s disease or other forms of dementia.
“The tools are here,” says Adrian Owen, a Professor of Cognitive Neuroscience and Imaging in the Departments of Physiology & Pharmacology and Psychology at Western University.
Ten years ago, Owen co-founded CIFAR’s Brain, Mind & Consciousness program, and he continues to serve as one of its two Co-Directors.
“Today, our ability to measure things far outstrips our ability to understand what we’re measuring. We can generate exquisitely detailed pictures of the brain in action, doing its thing. What we need to do now is learn how best to interpret that information.”
“Today, our ability to measure things far outstrips our ability to understand what we’re measuring. We can generate exquisitely detailed pictures of the brain in action, doing its thing. What we need to do now is learn how best to interpret that information.” — Adrian Owen
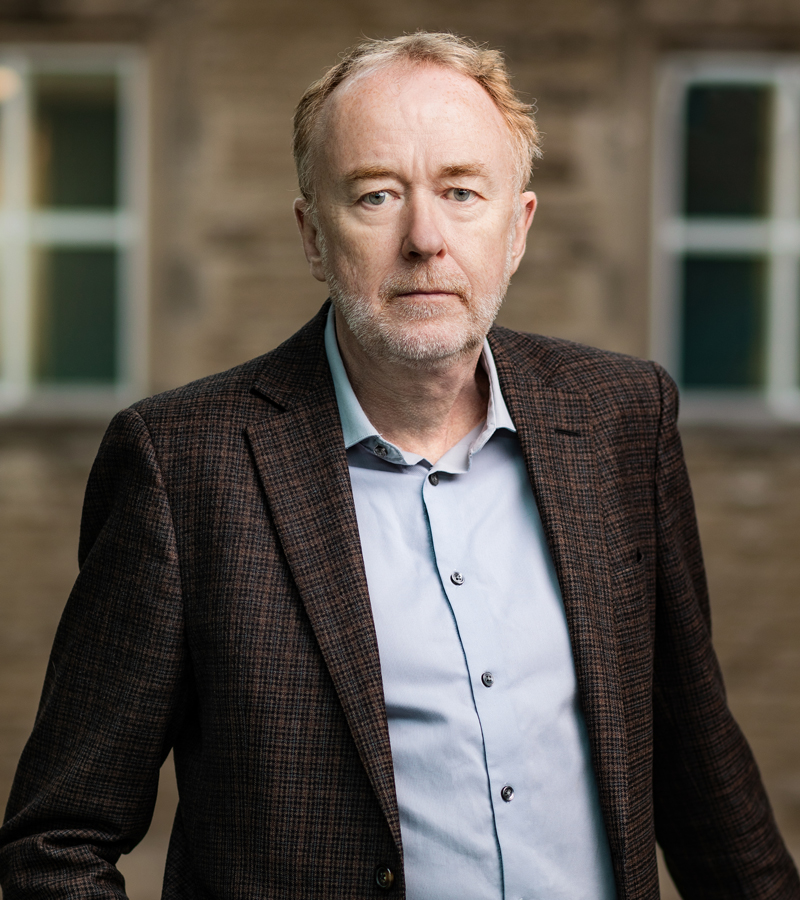
Photo: Derek O’Donnell
Every waking moment, something remarkable happens to you. Somehow, approximately three pounds of neurons, glia, proteins and other bits and pieces inside your skull produce the thoughts, feelings, memories and actions that define who you are.
How exactly physical matter gives rise to consciousness — often called the “mind-body problem” — is a long-standing mystery of both philosophy and science. It’s hard to assess how close we are to solving it, but what is clear is that new techniques and strategies are giving rise to a particularly rich period in consciousness research.
This research opens pathways to understanding cognitive functions, as well as new potential treatments for cognitive disorders such as Alzheimer’s disease or other forms of dementia.
“The tools are here,” says Adrian Owen, a Professor of Cognitive Neuroscience and Imaging in the Departments of Physiology & Pharmacology and Psychology at Western University.
Ten years ago, Owen co-founded CIFAR’s Brain, Mind & Consciousness program, and he continues to serve as one of its two Co-Directors.
“Today, our ability to measure things far outstrips our ability to understand what we’re measuring. We can generate exquisitely detailed pictures of the brain in action, doing its thing. What we need to do now is learn how best to interpret that information.”
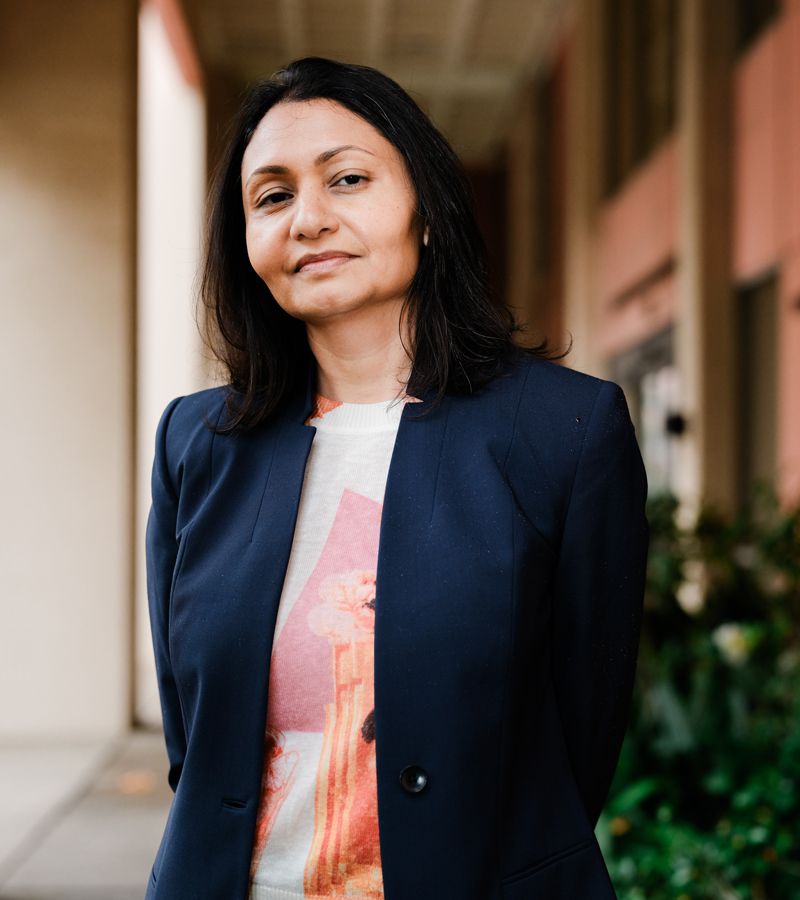
Photo: Christopher Brown
“For a long time, the thinking was that if a child is on the autism spectrum, we shouldn’t confuse them by also introducing two different languages too early. But more recently, research has indicated that bilingualism has no negative long-term effects on cognitive function.” — Lucina Uddin
How to image a brain
A century ago, German psychiatrist Hans Berger used a network of electrodes applied to the scalp to record the spontaneous electrical activity of a human brain for the first time. Known as electroencephalography (EEG), the method is still widely used in brain research.
Approximately 50 years later, EEG was joined by another technique that has since become just as central to the field: magnetic resonance imaging (MRI). Inside an MRI scanner, powerful magnetic fields are combined with radio waves to generate detailed images of structures inside the body. A modified version known as functional magnetic resonance imaging (fMRI) takes the process a step further, providing real-time information on how blood flow throughout the brain tracks with thoughts and behaviours from moment to moment.
By using blood flow as a proxy, neuroscientists can deduce which parts of the brain are most active when patients are completing a particular task or having a particular experience. Over the past few decades, they have built up libraries of EEG and fMRI images which can be analyzed to gain insights into how brain activity gives rise to cognitive function.
One well-known example comes from Owen himself. About 15 years ago, he and his team used fMRI images to show that some patients in so-called vegetative states can and do respond to external stimuli. In other words, they retain consciousness even if they aren’t capable of communicating it.
Since they first published their work, more than 1,300 patients in similar conditions have been scanned, with approximately one in five showing some level of consciousness.
“It’s now exploding in lots of other directions and I’m super happy to see people take this idea and run with it,” he says.
Tracking neural development and the “sense of self”
Today, fMRI scanners are more available than ever before and they are being applied to an ever-wider range of research questions.
Lucina Uddin is a CIFAR Azrieli Global Scholar in the Brain, Mind & Consciousness program. Her work traces the connections between brain activity and cognition in both typical and atypical development. For example, Uddin and her team have used fMRI to scan the brains of children between the ages of seven and 12, both with and without a diagnosis of autism spectrum disorder.
“What we see is that both groups of children are using the same regions of the brain to get a given task done,” says Uddin, a Professor in the Department of Psychiatry and Biobehavioral Sciences at the University of California, Los Angeles.
“But the sequence and timing of events might be different between the two groups. In other words, they are using different strategies to attain the same goal.” In a forthcoming study, Uddin plans to look at children raised in bilingual households versus monolingual ones.
“For a long time, the thinking was that if a child is on the autism spectrum, we shouldn’t confuse them by also introducing two different languages too early,” she says.
“But more recently, research has indicated that bilingualism has no negative long-term effects on cognitive function. If anything, it might be beneficial, for example, it may help with flexibly switching between different tasks.”
The ability of tools like EEG and fMRI to visualize the different mental mechanisms employed by patients to accomplish a certain task can also help make sense of conditions that impair consciousness, such as dementia and brain injuries.
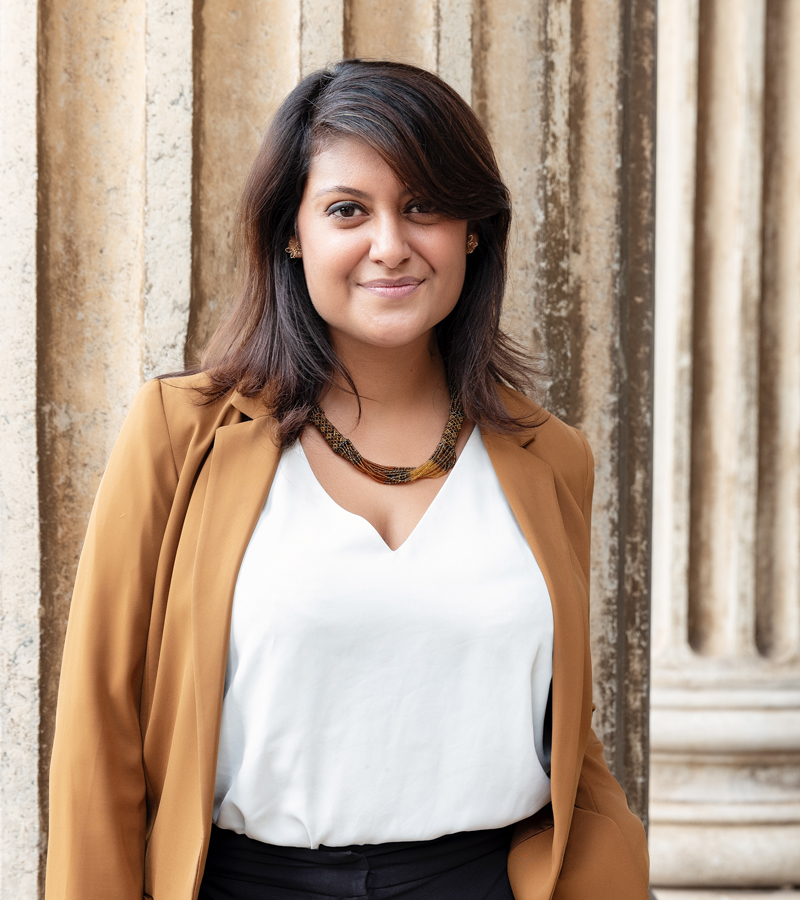
Photo: Bridget Corke
“The effect was subtle, and temporary, but it shows that we are not just brains moving around in the world... We relate to each other through our minds, through emotion, but also through our bodies.” — Sahba Besharati
Sahba Besharati, a CIFAR Azrieli Global Scholar in the Brain, Mind & Consciousness program, is addressing one of the most enduring mysteries of consciousness: how we develop self-awareness.
“As social animals, humans have a strongly developed sense of who we are, which in turn enables us to relate to others,” says Besharati, who is a Senior Lecturer in cognitive neuroscience in the Department of Psychology at the University of the Witwatersrand in Johannesburg, South Africa.
“One of the challenges people face when dealing with a cognitive disorder is experiencing a loss of this sense of self.” Besharati often works with patients who have experienced a brain injury — a stroke, for example — in which the damage is localized to the right hemisphere of the brain.
Some of these patients experience a condition known as anosognosia, which leaves them unaware of their
own neurological deficit. Despite being paralyzed on the left side, they might insist that they can move after all.
They may also confabulate, that is, create stories to explain why they are not moving. In previous work, Besharati
conducted a study in which these patients presenting with motor unawareness were given negative feedback on a behavioural task. To her surprise, these patients demonstrated elevated awareness of their paralyzed condition through the induction of negative emotion.
“The effect was subtle and temporary, but it shows that we are not just brains moving around in the world,” she says. “We relate to each other through our minds, through emotion, but also through our bodies.”
“What we find is that if we stimulate the right cells, we can get the learned behaviour to come back ... It’s changed how we understand aspects of memory coding and recall. It’s not that the memories have been lost; they’re still there.” — Tomás Ryan
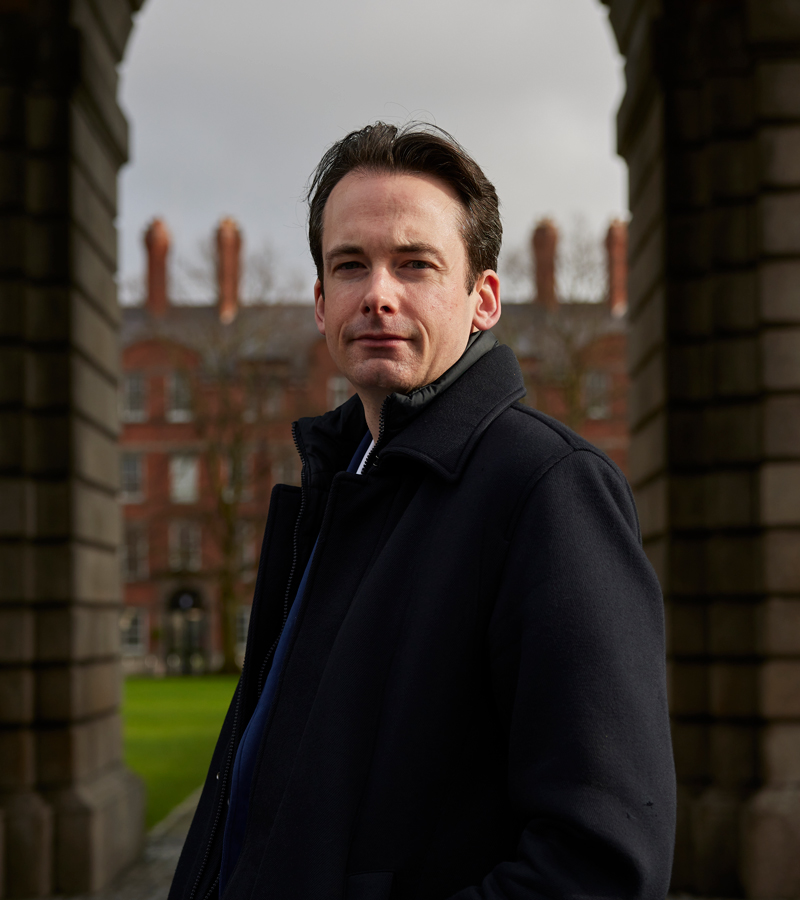
Photo: Mark Duggan
Tracing memory
Another mystery embedded in the mind-body problem is related to how information such as memories are encoded in the brain’s physical structure.
In the same way that scientists used the concept of genes to explain heritable traits long before the genetic code of DNA was deciphered, neuroscientists use the term “engrams” to describe units of cognitive information that can be stored, then later accessed and modified.
While we have yet to fully describe the physical basis of engrams, a new technique known as optogenetics is shedding light on the problem.
“What we’ve been doing over the last 10 years or so is developing methods that allow us to tag particular cells in the brain that are contributing to specific engrams,” says Tomás Ryan, a CIFAR Azrieli Global Scholar in the Child & Brain Development program.
“For example, by co-opting genes whose expression is a function of neuronal activity to express fluorescent proteins, we can see when that modified cell is firing,” says Ryan, an Associate Professor at the Trinity Institute of Neurosciences at Trinity College Dublin in Ireland.
“We can also express optogenetic receptors, which enable us to do the opposite: if we shine light on these cells, they will start spiking artificially.”
Using optogenetically modified mice, Ryan and his team can probe the process of forgetting, both the kind that happens naturally, as well as analogues of unnatural, pathological processes, such as traumatic brain injury or dementia.
After the mice have forgotten a particular behaviour, the team can stimulate cells that they know were related to the original memory.
“What we find is that if we stimulate the right cells, we can get the learned behaviour to come back,” says Ryan.
“It’s changed how we understand aspects of memory coding and recall. It’s not that the memories have been lost; they’re still there. Rather, at the moment you’re trying to recall them, you get a different one than the one you wanted. It’s a retrieval deficit, rather than a storage deficit.”
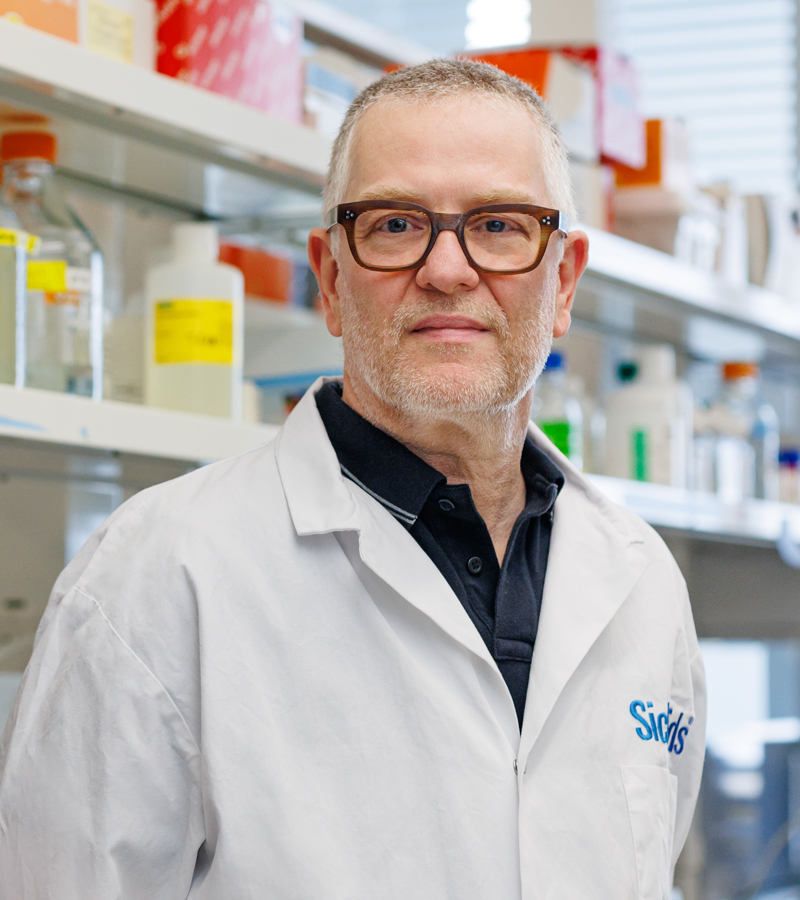
Photo: Josh Fee
“In some cognitive disorders, it may be that you have too little forgetting, and instead you get too many active memories interfering with the target one you’re trying to recall ... The brain isn’t like a hard drive on a computer, it doesn’t get full.” — Paul Frankland
Another researcher using optogenetics to study memory is Paul Frankland, a Fellow in the Child & Brain Development program and a Senior Scientist in the Neurosciences & Mental Health Program at SickKids Research Institute in Toronto, Canada.
Frankland and his team are particularly focused on a phenomenon that complicates our understanding of how memories are laid down and retrieved: neurogenesis, or the growth of new brain cells.
“You might think that if you have fresh new neurons, they could encode new memories more efficiently,” says Frankland.
“But the flip side of that is that you’re integrating those new neurons into stable circuits, so you’re potentially sort of overriding information that’s already been stored.”
This may help explain why memories created in infancy, the period where human neurogenesis is most strongly concentrated, are among the hardest for us to recall later in life.
In animal studies, Frankland and his team have artificially raised the rate of neurogenesis in mice and found that it did make them more forgetful. The degree to which humans continue to add new neurons throughout their lives is still debated by neuroscientists and at any rate, disorders such as dementia and Alzheimer’s disease are typically linked to a loss of neurons rather than the growth of new ones.
But Frankland says new paradigms of forgetting can offer valuable insights into both cognitive disorders and consciousness itself.
“Colloquially, and even among neuroscientists, we’re biased to think that forgetting is bad,” says Frankland. “But you can easily see how it can be beneficial: if you’re trying to remember where you parked your car today, it doesn’t help to remember instead where you parked it last week or last month.”
“In some cognitive disorders, it may be that you have too little forgetting and instead you get too many active memories interfering with the target one you’re trying to recall.”
New analogies can also help us think differently about the role that forgetfulness plays in consciousness, says Ryan. “The brain isn’t like a hard drive on a computer, it doesn’t get full,” he says. “I think of it more like a sculpture. You might have more or less material on it, but how much information that actually encodes is subjective.”
“As we accumulate more information, as the sculpture evolves, what you don’t want is to fixate on the first experiences you laid down. You want to be able to generalize, to make sense of the world. I think that’s part of the reason why we forget specific details.”
“You had something like 3 billion people under government-issued orders to restrict their social interactions, over a time span of weeks and months. What happens to the brain if you take that away?” — Danilo Bzdok
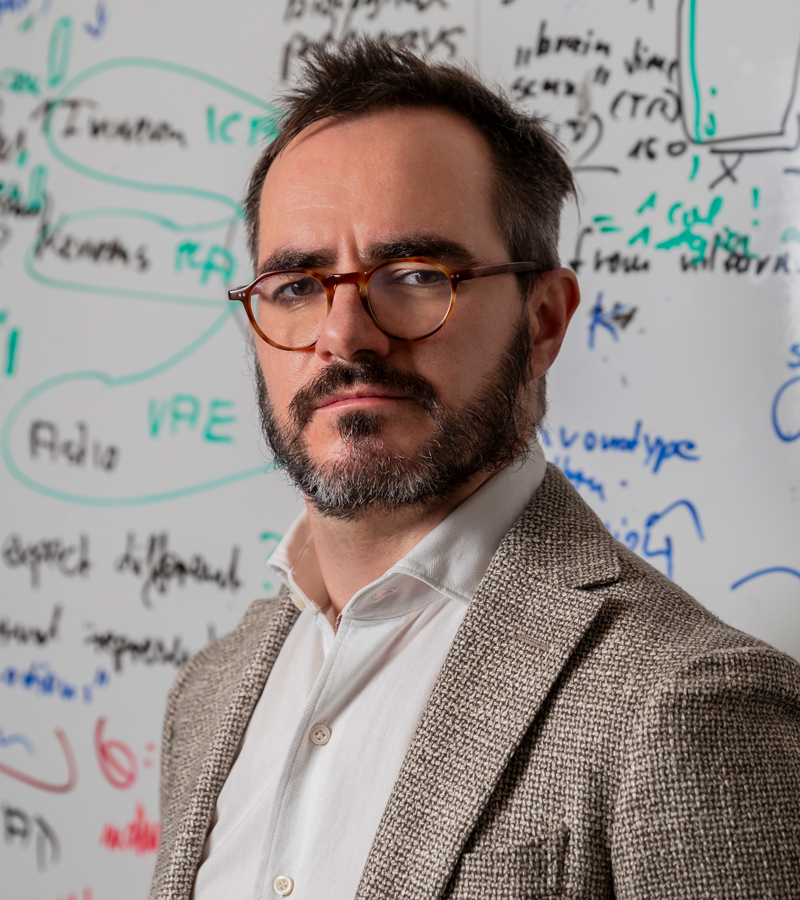
Photo: Dominic Blewett
Neuroscience in the age of big data
One of the newest and most promising tools in the study of consciousness has nothing to do with imaging the brain or manipulating its cells. Instead, it relates to analyzing the increasingly large amount of data that has been generated by existing methods.
“Over the last five to 10 years, neuroscience has entered the age of big data,” says Danilo Bzdok, a Canada CIFAR AI Chair at Mila, IVADO Scientific Co-Director and an Associate Professor in the Department of Biomedical Engineering and School of Computer Science at McGill University in Montreal, Canada.
“We are now capable of generating extremely large datasets of brain assessments and other medical information. It’s difficult to make use of these rich resources without resorting to machine learning or other forms of artificial intelligence.”
As both a neuroscientist and a computer scientist, Bzdok is uniquely positioned to leverage AI to analyze datasets that are simply too large for humans to handle, looking for patterns that have previously gone unnoticed. In fact, many of the datasets that he and his team focus on are the largest of their kind ever assembled.
One recent insight relates to the study of social isolation, a topic thrown into sharp relief by the lockdowns associated with the COVID-19 pandemic.
“We interpreted COVID-19 as a natural experiment at planetary scale,” says Bzdok. “You had something like 3 billion people under government-issued orders to restrict their social interactions, over a time span of weeks and months. What happens to the brain if you take that away?”
By analyzing brain recordings of 40,000 individuals and other phenotypes indexing everyday life, the team found something new: a number of changes in an area of the brain known as the default mode network.
Comparisons of human brains with those of other animals suggest that the default mode network was added relatively recently in our evolutionary history. As such, it may be responsible for some of our unique social behaviour, in addition to other human-defining mental capacities.
“This brain region is very metabolically expensive; it uses a lot of energy,” says Bzdok.
“It’s also the part that remains malleable the longest in our development and now we know that it’s also where social isolation takes its toll. It’s something that hadn’t been reported in previous studies of loneliness.”
Analyzing large datasets with AI could accelerate research on many fronts. For example, it could help identify previously unrecognized risk factors for conditions such as dementia and Alzheimer’s disease, or point to traits or therapies that might slow the decline associated with these conditions.
Yet another example of the “big data” approach to neuroscience comes from Owen and his team.
“Last year, we published a study in which we went back to fMRI data we had collected 10 years previously. It was what’s called resting state data, from patients in a coma,” he says.
“Back then, the best we could do was compare those images to healthy patients to try and guess which ones would be the most likely to recover.”
“But one of my smart graduate students was able to design a machine learning algorithm for this task. We found that it could predict with 80 per cent accuracy who would recover and who wouldn’t. I think that shows you where the field is going.”
Owen is optimistic about the potential of AI and machine learning to help neuroscientists make sense of their ever-growing collections of data. But he also points out that the very term “artificial intelligence” itself complicates our understanding of consciousness.
“For the longest time, people relied on concepts like the Turing test as a way of determining whether or not something is conscious,” says Owen.
“Well, we now have lots of AI systems that can pass the Turing test with flying colours. And at the same time, most toddlers wouldn’t be able to pass it. Now, I don’t think you need to accept that machines are conscious or that toddlers are not, but I do think it points to the need for a new test.”
The fact that it’s still not easy to define what consciousness is underscores the challenges facing those who seek to understand how it arises. But the scientific advances being led by CIFAR researchers show that global collaborations from diverse fields, including neuroscience, psychology and computer science, are accelerating progress.
By working together, we can not only help those who need it, we can also learn more about what makes us human.
Related Articles
-
Nicolas Papernot and Catherine Régis appointed Co-Directors of the CAISI Research Program at CIFAR
December 12, 2024
-
CIFAR signs San Francisco Declaration on Research Assessment
December 09, 2024
-
Graham Taylor, Vector Institute: Using AI to track insect biodiversity loss in real time
November 29, 2024
-
Ross Mitchell, Amii: AI note-taking for doctors
November 16, 2024